Sourcing Silicone Parts: Injection Mold or 3D Print?
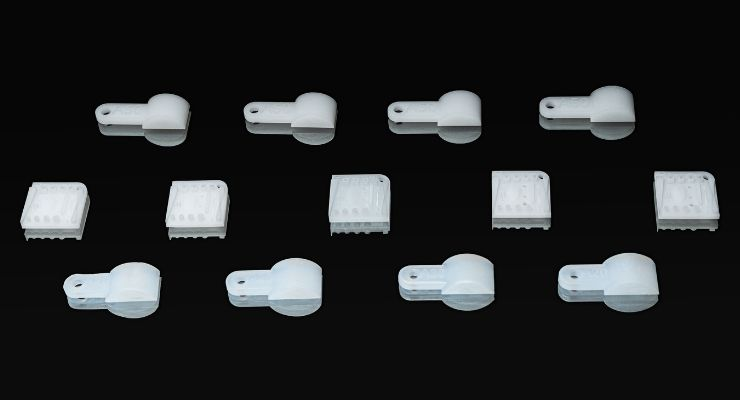
Medical device manufacturers have plenty of materials to choose from when designing parts, but one of the most popular and widely used is liquid silicone rubber (LSR), more commonly and somewhat inaccurately referred to as silicone. That’s because this versatile material is durable yet flexible, soft to the touch, and above all, biocompatible (assuming it’s a medical-grade LSR), making it the first choice for a wide variety of components.
We’ll dive more deeply into the specifics of LSR shortly, but top of mind for many designers is whether they should plan on plastic injection molding their silicone parts or is rapid prototyping, a.k.a. 3D printing, a possibility.
As with so many other manufacturing-related questions, the answer depends on numerous factors, among them production quantity, part geometry, accuracy and surface finish requirements, and much more. And as you’ll see, true silicone printing has only recently become a reality, with practically all prototypes (until now) made of alternative materials.
In this article, we’ll explore the pros and cons of each manufacturing approach and attempt to provide some guidance as to which one makes the most sense for a given application. We’ll also introduce a relative newcomer to the 3D printing world known as silicone additive manufacturing, or SAM, and offer some information on its use. Read on.
A Real Toy Story
Silicone is a synthetic polymer that scientists know as siloxane. It consists of long chains of oxygen and silicon surrounded by groups of carbon, hydrogen, and other elements, which vary based on the type and grade of silicone. Its roots go back to 1823, when Swedish chemist Jöns Jackob Berzelius isolated silicon—the stuff that makes up today’s semiconductors—and then went on to create silicon tetrachloride, a compound used in silicone production.
Some credit Englishman Frederic Kipping as silicone’s inventor, and he reportedly coined the term in 1901, although most in the industry name American James Franklin Hyde as the “Father of Silicones.” Regardless, silicone wouldn’t take off (quite literally) until World War II, after the fledgling Dow Corning company (where Hyde worked) developed a silicone-based grease for use in high-altitude Allied aircraft.
The first commercial application for silicone was in Silly Putty, which seems kind of incredible today, but it quickly became a go-to material for a wide range of industrial uses. Automakers began using it in gaskets and to wrap electrical wires, construction companies found it effective for adhesives and sealants, and NASA even gave Neil Armstrong a pair of silicone-soled boots for his infamous stroll on the moon. Today, silicone exists in everything from antacids and brake fluids to cookware and cell phone cases, with more uses coming online each day.
Making the Grade
In the medical world, LSR is especially ubiquitous. Thanks to its flexibility, resistance to heat and chemicals, and the aforementioned biocompatibility, medical-grade silicone has long been used for prosthetic devices such as breast and ear implants, as well as orthotics like braces and arch supports. LSR is usually the first choice for intravenous tubing, catheters and shunts, syringes, breathing tubes, and insulin pumps. Wound dressings frequently contain silicone, as do the valve seals and gaskets in medical equipment. Silicone rubber is also quite suitable for wearables and other consumer products—watch bands, for instance, or the soft, squishy covers seen on earbuds and hearing aids. It’s everywhere.
What separates medical-grade LSR from other types of silicone? Not all that much, actually, with the primary difference coming down to how the material is cured during or immediately after the manufacturing process. For instance, commercial LSR employs hydrogen peroxide and high temperatures to permanently cross-link silicone’s polymer chains, which leaves behind acid residues and other by-products that may cause it to fail biocompatibility testing.
Medical LSR, on the other hand, relies on a platinum-based catalyst to cure the LSR, followed by a secondary “baking” or UV-light treatment (depending on the application). This procedure eliminates harmful by-products and makes the product safe for use on, in, or around the human body, although sterilization is still required for most applications.
That last statement is critical. Medical device manufacturers must specify the type of human contact and how long their products will be used if they’re to gain FDA approval. Direct contact is exactly what its name describes but is further defined as limited, prolonged, or permanent. There are also indirect, transient, and non-contact usage levels, all of which are outlined under ISO 10993-1: 2018 and other international standards.
Making Parts
There’s much more to the science of LSR and the qualification steps necessary for any medical part development project, whatever the materials. For now, though, let’s discuss the manufacturing process. As indicated earlier, plastic injection molding is the most common method for making LSR parts, and for good reason. It’s fast, accurate, capable of producing the fine finishes common to medical parts, and is very cost-effective in higher production quantities. And there’s the rub.
Anyone who’s ordered injection-molded parts knows that the tooling can be quite expensive. It can also take months to deliver said molds, making time to market a serious constraint. Fortunately, a number of online manufacturing companies, including Protolabs, now offer “quick turn” injection molds machined out of aluminum rather than hardened steel. In most cases, these can be produced within a week or so, making even small quantity jobs both fast and affordable.
Still, some medical device firms would prefer to 3D-print their prototype and low-volume production parts. Who can blame them? Additive manufacturing (AM) delivers high-quality, end-use components in days and sometimes hours, allowing designers to make multiple design iterations quickly. It also provides greater design freedom than traditional processes, with none of the concerns over flash, voids, and part ejection that can occur with injection molding.
There’s just one thing: LSR is a problem child for most 3D printing technologies. Between its relatively high viscosity and somewhat finicky curing requirements, accurate layer-by-layer deposition has proven difficult to achieve.
It’s for this reason that manufacturers have turned to silicone-like polymers such as thermoplastic polyurethane (TPU) and thermoplastic elastomer (TPE) as alternatives to LSR. There are also a number of proprietary stereolithography resins available that mimic silicone’s properties and, like TPE and TPU, are much less expensive than LSR. All of these materials come close in terms of flexibility, tear strength, biocompatibility, and other medically desirable properties, but they are not LSR.
Meet SAM
Before discussing the one viable option for true 3D-printed LSR, it’s important to point out an alternative, which is to 3D-print tools for LSR molding. Doing so eliminates the need for “hard” molds and, since they can often be produced more quickly than molds machined out of aluminum, are an attractive option. Buyers should be aware, however, that these soft tools are just that—softer than metal—and therefore offer limited accuracy and production life.
Which brings us to SAM, short for silicone additive manufacturing. Developed by Spectroplast AG of Switzerland, SAM is the only 3D printing technology able to build parts from medical-grade silicone, which the company has dubbed TrueSil. It provides excellent feature detail and surface finish, with resolution and geometric freedom comparable to that of conventional stereolithography.
According to the manufacturer, SAM is a light-based system that cures a full layer of silicone parts in a single exposure. This means the number of parts does not affect print time as with competing systems, and by packing parts more densely on the build plate, production costs are often lower than other 3D printing processes. Part sizes are currently limited to “about the size of a smartphone,” although the company will soon introduce a machine with a significantly larger footprint.
At least one well-known U.S. service bureau has recognized SAM’s game-changing capabilities and added it to their other 3D printing (and injection molding) offerings. The new technology has enabled the company to provide its customers with silicone components in translucent white and black with durometers ranging from 20-60A, comparable to injection-molded LSR parts.
SAM is also said to generate smoother finishes than competing AM technologies. For example, parts made from TPU using selective laser sintering (SLS) have a somewhat rough texture, similar to that of a sugar cube, and are limited to white, although they do boast excellent biocompatibility.
Polyjet, on the other hand, generates smooth finishes, albeit not as fine and layer-free as seen with SAM parts. It also offers color choices and durometer levels similar to those available with SAM, but the digital photopolymers are only suitable for prototyping and form/fit/function testing, as they are not deemed biocompatible. It should be noted that these alternative materials are less expensive than LSR, which might be a significant concern at higher production volumes or with large, bulky parts.
Choices, Choices
So, which is the best choice for your medical application—injection-molded LSR parts, or ones that are 3D-printed with SAM? As stated at the outset, the answer depends on numerous factors, but the good news is that product designers looking for the quickest turnaround possible no longer need to rely on alternative polymers that might not offer the same level of performance or part quality.
But even the most robust material will fail if the designer doesn’t follow the recommended guidelines for the intended manufacturing process. In SAM’s case, these are similar to most 3D printing technologies, with the chief concern being the need for temporary support structures to prevent curling and deformation on horizontal or near-horizontal surfaces. Tall, skinny walls can also be problematic, as are holes under 0.040 in. (1 mm) or so in diameter, particularly those with long aspect ratios.
Injection-molding LSR is fairly straightforward, although it is distinctly different than “regular” thermoplastic molding. The material flows freely, so thin walls are typically not a concern as with plastics like polyamide (nylon) and PEEK. Nor is “sink” (caused by overly thick sections) a problem, although flash at parting lines occurs more readily with LSR. Also be aware that LSR does not like sharp edges, which means that delicate parts especially can tear during removal from the mold, so internal corners should be rounded. Thanks to its flexibility, though, moderate undercuts and “bump off” features are easy to accommodate, and designers will be happy to know that injection-molded LSR can be made into a virtual rainbow of colors.
Peering Around the Corner
This is admittedly a 30,000-foot view of the best practices for each of these processes, which is why many online service bureaus provide manufacturability analyses to potential customers. What they might not tell medical part developers, however, is to avoid painting themselves into a 3D-printed corner. Silicone injection molding has few design constraints, but it does not compare to the freedom that comes with 3D printing.
Designers might therefore prototype a part using SAM or one of the other AM technologies mentioned earlier, only to find their parts are unmoldable once they’re ready to enter the high-volume commercial market. The message is clear: always evaluate designs for manufacturability at all stages of the product lifecycle, and always discuss future plans with your manufacturing partner early on. Doing so can avoid an expensive lesson later.
Manufacturing considerations aside, it’s an exciting time for any medical device designer who wants to take advantage of silicone’s many desirable qualities. Whether 3D-printed or injection-molded, this important elastomer repels water, has low thermal and electrical conductivity, is resistant to ultraviolet light, radiation, oxygen, and most chemicals, and—grade permitting—can be used safely for years and years within the human body. And thanks to the continuous forward march of advanced manufacturing, silicone can now be used to 3D-print a wide range of cost-effective medical parts boasting the fine finishes and accuracy normally associated with injection molding.
Article Source:MPO