Plastic Gears Are Enabling Today’s Advanced Applications
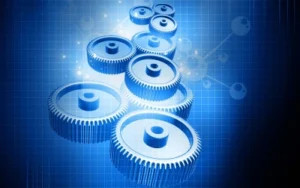
At a Glance
- Newer, highly engineered materials offer extended performance characteristics for demanding gear applications.
- Material and tooling costs for plastic gears are generally lower compared with the costs of metal gears.
- Before you make the switch, though, consider operating environments, resin properties, and manufacturing options.
Design engineers face continuous pressure to enhance product performance while driving down costs. For many devices, converting metal components to plastic is an effective way of achieving both goals. Gears are a great example of a metal-to-plastic conversion that can reap many benefits for both the design engineer and the end user.
In this article, we’ll take a look at some of the advancements that have enabled the conversion to plastic gears, the potential benefits, and the key design considerations, whether you’re converting metal gears from a legacy product or designing a novel device.
What Has Driven the Shift from Metal to Plastic?
There’s growing demand for high-performance gears to power some of the fastest-growing medical technology applications, including robotic-assisted and minimally invasive surgery (MIS) systems, drug-delivery devices such as infusion pumps and auto injectors, and wearable and connected devices that collect health and fitness data. In addition, plastic gears drive applications such as automation and motion control in demanding industries including automotive, aerospace, and HVAC.
Technology innovations have also propelled the shift to plastic gears. First, the options for polymer materials have advanced beyond the acetals, nylons, and polyesters that have commonly been used for gears for decades. Newer, highly engineered materials like Ultem, Radel, PEEK, and Delrin can offer extended performance characteristics. For example, PEEK can withstand the high temperatures of the autoclaving process. Additives such as glass and carbon fibers provide higher flexural strength and further bolster performance in demanding applications.
Second, new technologies have improved design for manufacturability (DFM) capabilities in the injection molding process. For example, we can now conduct FMEA on a design using software, rather than actually producing the part and performing benchtop testing such as strength and stress tests. With Moldflow simulation software, we can analyze and optimize designs for performance, quality, and cost before investing in tool fabrication. We use Moldflow Adviser to reveal air traps and weld lines, identify potential warpage, and provide guidance on mold filling, packing, and cooling, to name a few steps to optimize the design.
Third, advancements in measurement techniques such as Kistler sensors that monitor cavity pressure and temperature in the injection molding process have enabled better data collection and analysis. We’ve also seen advances like Zeiss Gear Pro software for Zeiss coordinate measuring machines (CMM) that enables us to measure and analyze all common types of gear teeth.
What Are the Potential Benefits of Plastic Gears?
Converting from metal to plastic gears can provide a wide range of benefits for design, manufacturing, and performance.
1. Cost Effectiveness & Speed to Market
Plastic gears generally offer both a lower material cost and a lower tooling cost compared with the costs of metal gears. The plastic injection molding process allows multicavity tools that enable more parts per shot, increasing throughput. In addition, an injection mold can be built for multiple processes, such as insert molding, overmolding, and two-shot molding. This streamlines the manufacturing process and eliminates the need for secondary operations such as finishing, plating, and machining, which saves both time and cost.
2. Performance
With the trend toward miniaturization in medical devices, lighter-weight plastic materials are an attractive choice, particularly for technology such as wearable drug-delivery devices. Weight is also a significant driver in the automotive industry, where design engineers are continuously challenged to reduce every gram of weight they can to meet tough fuel economy standards. Unlike their metal counterparts, plastic gears act as shock absorbers to mitigate noise and vibration. In addition, the inherent lubricity of plastic materials contributes to reduced friction for smoother actuation.
3. Quality & Reliability
As the backbone of gear production, the injection molding process provides built-in reliability and consistency. Injection-molded gears often meet specifications right out of the mold, minimizing the need for extensive post-processing or adjustments. Integrating other molding processes into the mold—for example, adding tabs or holes—reduces the risk of flaws and imperfections that can occur with secondary operations. The chemical, corrosion, and abrasion resistance of plastic gears further bolster their long-term reliability, ensuring consistent performance and longevity in demanding environments.
4. Flexibility of Design
The injection molding process offers unparalleled flexibility in gear design, including complex gear shapes, intricate geometries, and holes. With hundreds of thermoplastic materials at their disposal, design engineers can tailor gear design to meet the specific requirements of diverse applications.
Photo by FilmPublicityArchive/United Archives/Hulton Archive via Getty Images
What Are Some Key Design Considerations?
The primary driver of gear design, of course, is the application and its requirements. Here are some factors to consider when you’re converting from metal to plastic gears.
1. Environment
Environmental considerations include:
-
Thermal expansion. Plastic gears expand as temperatures rise more than metal gears do. It takes precise engineering to ensure that gear teeth engage and perform properly to accommodate thermal expansion. It’s also important to confirm that gears fit and function within the designated space despite temperature fluctuations.
-
Effects of moisture. Some types of gear resins can absorb moisture, causing dimensional changes. Gear design needs to take into account potential swelling in moist environments to ensure that gears have the space to engage properly.
-
Effects of chemicals, corrosion, and abrasion. Exposure to certain chemicals can cause resins to stress crack or erode, compromising performance and longevity. High temperatures can accelerate these effects. Gear resins must be carefully chosen to withstand the demands of the application environment.
2. Resin Choice
Selecting the right resin for the application is critical to the success of gear conversion. With options ranging from traditional acetals, nylons, and polyesters to highly engineered materials such as Ultem, Radel, and PEEK, engineers have a vast toolbox of resins to accomplish desired performance characteristics. Resin considerations include:
-
Weight. Does the application need to reduce weight, as in the case of injectables or wearables?
-
Strength. How does the material handle stress, load, or torque?
-
Lubricity/surface finish. Does the application require low friction?
-
Temperature fluctuation. Does the gear need to withstand heat, such as the autoclaving process?
-
Fatigue life. How long will a material last in the application?
-
Interaction with chemicals. Will the material withstand exposure to chemicals in the environment?
3. Manufacturing Methods
Various injection molding operations—such as insert molding, overmolding, or two-shot molding—may be required to manufacture complex gear designs efficiently and cost effectively. Secondary machining operations may be necessary for integrating components such stainless-steel pins or bushings.
4. Cost Targets
Design engineers are continually facing pressure to reduce cost while maintaining quality. Injection molding is a highly cost-effective process. Once you’ve invested in the mold, you can make parts relatively inexpensively. The type of gear you choose can also influence the cost of the mold. For example, while standard spur gears are simpler and less expensive to produce, a helical gear may be more appropriate for the application and more cost-effective in the long run, despite the higher upfront cost.
How Do I Get Started?
Design engineers considering a conversion from metal to plastic gears or designing a novel geared application should look for a partner with solid experience in resin selection, optimizing mechanical gear performance, and mesh analysis, including backlash, pressure angle, sideroll ratio, root clearance, and contact ratio to optimize gear performance.
It’s important to work with a partner that understands the intricacies of designing and manufacturing plastic gears for advanced life science applications, motion control, and automation. Considerations should include not just cost, but the gear environment, resin choice, and manufacturing method to best meet the needs of the application.
By leveraging the cost-effectiveness, performance, quality, and design flexibility of plastic gears, design engineers can better meet the demands of increasingly complex, technology-driven innovations.
Article source: MDDI